Pharmacogenomics: A Review of its Objectives, Successes, and Limitations
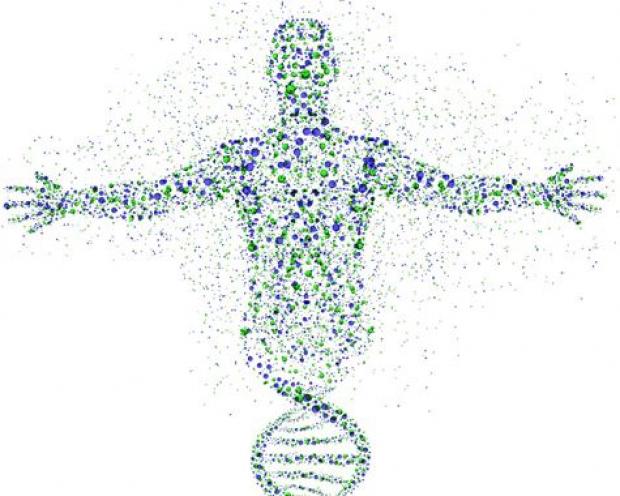
Abstract
Pharmacogenomics is the study of how a person’s genes affects their body’s response to drugs. While current medical drug therapies tend to treat all patients as if they were the same, differences in one’s genome can determine whether a treatment will be effective, ineffective, or even harmful. Yet despite the research supporting pharmacogenomics and its impact on human health, patient-tailored therapies are not yet widespread in clinical settings. To understand why, Hannah Shaw explores the impact of pharmacogenomic research and the reasons why its influence is still limited.
I. Introduction
No two people are exactly alike. So why should doctors treat diseases as if every patient were the same? The field of pharmacogenomics aims to change that. Pharmacogenomics, also known as personalized medicine, is the study of how genetic variations in a person’s genome affect their response to drug therapies. It aims to develop safer, more effective drug therapies by tailoring medications to a patient’s genome (Sadée and Dai 2005, p. 243). By sequencing a person’s genes and finding specific variations, doctors can predict how the patient will respond to a drug and adjust their prescription as necessary. The widespread adoption of pharmacogenomics in clinical settings will lead to more effective drug therapies for patients suffering from ailments ranging from cancer to depression, as doctors could tailor prescriptions to their patients’ genomes to deliver targeted therapies.
Scientists have already found connections between genetic variation at particular genes and patient responses to cancer-treating drugs, blood-thinning drugs, and more. Other researchers have linked most Adverse Drug Reactions (ADRs) to changes in metabolism caused by genetic variations, and that pharmacogenomics can predict and avoid most of these ADRs (Soto et al. 2012, p. 11). This research shows that genetic variation does play a role in drug response, and doctors have used this information to deliver safer and more effective drug treatments for a variety of ailments. Scientists are currently researching whether mutations at certain genes will help better understand how other diseases with those mutations will respond to treatment.
Yet despite the great potential of pharmacogenomics, genetic analysis is not a common practice in most clinical settings. The reasons for this are many: lack of pharmacogenomic education among the public, cost, accessibility, lack of research, regulation from lawmakers, and privacy issues surrounding patients’ genetic information (Centre for Genetics Education 2015, p. 3). Though the obstacles preventing pharmacogenomics from being more widespread are many, its benefits and potential warrant further research and more creatively analyzed data in the field.
The purpose of my research is to investigate the role of pharmacogenomics in drug therapies and clinical settings. To that end, I will conduct a literature review to discuss what pharmacogenomics is, how it works, its successes, its limitations, and why it has not been more widespread in clinical settings despite all its benefits. Pharmacogenomics is a promising field that has already made great contributions to medicine and drug therapies, and it has the potential to improve drug treatments even further.
II. Applications and Benefits
The field of pharmacogenomics offers potential in a wide range of medical disciplines, and has already seen several successes. Scientists have already found that mutations in certain genes can affect how patients respond to specific drugs. For example, researchers have discovered an association between mutations in a gene called CYP2C9 and the blood-thinning drug warfarin. People with a particular mutation in this gene exhibited an increased risk of bleeding out due to warfarin (Higashi et al. 2002, p. 5). Other researchers have found connections between mutations in a gene that controls metabolism called CYP2D6 and patient response to opioids and antidepressants (Ko et al. 2016, p. 2).
Aside from these medications, researchers have been investigating pharmacogenomic interactions in a variety of different diseases, including heart disease, stroke, and depression. One disease that has seen the most results and the most benefits from pharmacogenomics is cancer. Scientists have discovered that mutations in certain genes can affect how different cancers respond to treatment from cancer drugs. For example, researchers have discovered many single nucleotide polymorphisms (SNPs), variations in a single base pair in a DNA sequence that lead to breast cancer (Sauna et al. 2007, p. 1), and can prescribe the best treatment based on the presence of these genetic markers. Other studies have shown that the presence or absence of estrogen receptors in breast cancer cells, receptors that bind to the hormone estrogen, can determine whether the tumor will respond to the drug most used to treat breast cancer (Sauna et al. 2007, p. 3). Genetic analysis can detect these receptors, so doctors have been using pharmacogenomics to determine whether patients with breast cancer should use the most popular drug or start with a different drug that is more likely to work. Through these studies and many others, doctors have developed better drug therapies and treatment plans for patients with different genetic variants of cancer, improving success rates, while also reducing the negative side effects of these drugs (McLeod 2013, p. 2).
Pharmacogenomics has also led to economic benefits as well, as patients can determine which treatment will work best for them based on genetic analysis of their disease, instead of trying a series of drugs before finding one that worked for them. Thus, through pharmacogenomics cancer patients save themselves a lot of time and money, while sparing themselves the harmful side effects of taking ineffective drugs. These are a few examples of how pharmacogenomics have improved cancer treatments, and researchers are looking to replicate these successes in other diseases and medical disciplines as well.
Aside from increasing the effectiveness of drug therapies, pharmacogenomics is also important in avoiding Adverse Drug Reactions (ADRs). ADRs are far worse than the typical side effects one expects from medication. There are over 2 million serious ADRs per year; in the United States ADRs are the fourth leading cause of death, causing 100,000 deaths yearly (CERT 2018). If doctors and regulators decide that the risks outweigh the benefits due to too many deaths or illnesses, the market will withdraw drugs (Soto et al. 2012, p. 12).
Polymorphic enzymes metabolize, or break down in the body, fifty-nine percent of all the drugs that cause ADRs (Soto et al. 2012, p. 14). A genetic mutation or natural selection modify a polymorphic enzyme’s amino acid sequences or composition; though, it is still able to catalyze the same chemical reaction as a non-mutated version of that enzyme. Genetic variations in a person’s genome and enzymes can also interfere with the metabolic pathways that the body uses to modify drug substances, and this interference accounts for the most common and severe ADRs (Soto et al. 2012, p. 14).
Traditionally, doctors would not know if a drug would cause ADRs in patients until after the drug was prescribed, resulting in patients being hospitalized or even dying from ADRs that were preventable. Yet, with the advances in pharmacogenomic research and technology, doctors can now analyze a patient’s genetic sequence for any variations that could cause ADRs for a specific drug before writing a prescription. If they find that the patient has genetic variations that would result in ADRs, the doctors can adjust the dosage or prescribe a different drug that would not induce any ADRs. Thus, not only can pharmacogenomics improve a drug’s success rate, it can also mitigate or even eliminate any harmful failures as well.
III. Obstacles
Despite these successes, clinical settings do not commonly implement pharmacogenomics. As noted, there are several reasons for this (Hamburg and Collins 2010, p. 3). One reason is that there are so many possible gene and drug interactions, many of them complex due to involving multi-step pathways and several different proteins. These pathways, along with the sheer number of genes in the human genome, make it difficult for researchers to determine which genetic variations in a gene are medically relevant enough to be worth pursuing (Katsios and Roukos 2010, p. 4). There is also the debate of whether the effects of genetic variation on drug therapies are significant compared to other factors, such as lifestyle, environment, and interactions between different drugs.
Aside from the scientific challenges, there are other obstacles preventing pharmacogenomics from being more widespread. One such obstacle is cost. Despite advances in genetic sequencing technology making genome analysis cheaper and easier, genetic analysis tests can still be quite expensive, and insurance companies are hesitant to cover the costs of these tests due to lack of data on pharmacogenomics’ cost-effectiveness (Swen et al. 2007, p. 2).
Another issue is accessibility. Most clinics do not have a genetics expert on call, requiring them to send patient results out of clinic, adding both time and cost to the genetic testing. In addition, many primary care doctors are not fully qualified to explain the full impact of a patient’s genetics on disease and treatment, so when patients ask their doctors questions about genetics, the doctors are not able to answer all of their questions (Kitzmiller et al. 2011, p. 1).
Another issue is that the average person does not know much about pharmacogenomics, which hinders the implementation of pharmacogenomics as studies have linked a lack of education to less support for personalized medicine (Nielsen and Møldrip 2006, p. 4). Research has shown factors such as race, gender, socioeconomic status, and nationality affect how likely a person is to support implementing pharmacogenomics into the clinical setting (Nielsen and Møldrip 2006, p. 6). For example, researchers have found that Caucasians tend to be more restrictive towards implementing pharmacogenomic data into the healthcare sector, while Hispanics were less concerned (Nielsen and Møldrip 2006, p. 4). They also discovered that while young people were the most concerned about the misuse of private genetic data, they were also the most interested in submitting to genetic testing (Nielsen and Møldrip 2006, p. 5).
There is also the matter of regulating pharmacogenomics, as the field advances faster than lawmakers create laws and guidelines to govern it (Hamburg and Collins 2010, p. 1). The fact that most policymakers are not deeply educated on genetics creates the issue where they are creating regulations for technologies they do not understand. More importantly, people are concerned with creating regulations that balance both patient privacy and the need for researchers to access data for clinical trials and databases (McLeod 2013, p. 3). Many people worry about protecting the privacy of their genetic data, as they fear what the consequences could be if their employers learned this data (Nielsen and Møldrip 2006, p. 4). These issues are the biggest obstacles preventing pharmacogenomics from seeing greater use in the clinical setting.
IV. Possible Solutions
While these challenges are formidable, they are solvable. There are already several researchers working to address the scientific obstacles by innovating methods of investigating the impact of genetic variation on drug effectiveness and creating online databases where anyone can search for hundreds of known gene mutation and drug interactions (PharmGKB 2018) and over 700 ongoing or completed pharmacogenomic studies (Nat Library Med at NIH 2017). Researchers are investigating ways to execute pharmacogenomics better by implementing personalized medicine programs in pharmacies and nursing homes (Nat Library Med at NIH 2017). Other researchers have recognized important attributes that SNPs must have in order to have major clinical importance. This includes a dramatic effect on the total concentrations of all of the active components of a drug, a clear association of concentration with desired effect, and significant severe concentration-related adverse effects to the drug. Another attribute includes the drug in question having a low therapeutic index, meaning there is a low ratio between a drug’s lethal dosage and the amount of dosage that causes a therapeutic effect (Gardiner and Begg 2006, p. 1). These attributes create guidelines for determining which genetic mutations have major clinical importance and merit further investigation, while researchers can use these guidelines along with their colleagues’ data to steer their own studies. Researchers can then record their findings in online databases for other scientists to review and expand upon, thus encouraging and innovating further research in the field of pharmacogenomics.
While I do not claim that I have the answers to all of the other obstacles, based on my research I can suggest possible solutions for some of them. For one, I would recommend training primary care doctors such as family doctors in genetics, so that they would be able to address any concerns their patients have about genetics and how that affects their disease and treatment (Kitzmiller et al. 2011, p. 1). While the cost to train these doctors would be high, I would argue that receiving better, more informed healthcare would help people protect their health, as well as decrease the severity and duration for which they are sick. This, in turn, would save money that otherwise would have been spent on medical bills (Tollman et al. 2001, p. 37). As all health insurance companies know, it is more cost-effective to spend a dime preventing illness than to spend a dollar treating it.
We must take greater measures to educate the public in pharmacogenomics, in order to foster greater understanding and appreciation for how our genes play a role in our health. Research has shown that people who are educated on pharmacogenomics and its capabilities are more accepting of it, which would increase the possibility for the successful implementation of pharmacogenomics in the clinical setting (Nielsen and Møldrip 2006, p. 6). Educating primary-care doctors and nurses deserves a special emphasis, since people will turn to their doctors with questions if they do not understand how pharmacogenomics affects their health and treatment. As a bonus, greater awareness of pharmacogenomics would generate more interest and support for pharmacogenomics, pushing lawmakers to channel more funding into pharmacogenomic research.
V. Counterarguments
Critics of personalized medicine may argue that these solutions are insufficient towards solving the issues preventing pharmacogenomics from seeing wider clinical use. Detractors of pharmacogenomics may utilize the lack of research for pharmacogenomics in defense of diverting resources to developing and improving known effective treatments (Kimmel et al. 2013, p. 8). However, the lack of research on a particular topic has never been an excuse for less research. Instead, there not being enough research in a field has only spurred scientists to conduct their own investigations, and pharmacogenomics is no exception. Another point to note is that pharmacogenomics is most certainly not lacking in research. According to the online database of the U.S. National Library of Medicine, there are over 700 completed or ongoing studies related to pharmacogenomics and its applications.
Another argument is that the cost of developing drugs personalized for a specific genetic population is too expensive to make pharmacogenomics feasible on a wider scale (Kimmel et al. 2013, p. 8; Swen et al. 2007, p. 3; McLeod 2013, p. 3). However, an economic review conducted in 2001 showed that through the successful application of pharmacogenomics, the improvements in efficiency and success rates would save pharmaceutical companies more than half a billion dollars per drug (Tollman et al. 2001, p. 12). Despite the potentially smaller market for a personalized drug, pharmacogenomics could lead to a net increase in profits for drug companies due to greater rates of adoption for the drug, identification of patients who otherwise would not have been candidates for the drug, increased compliance due to improved effectiveness and premium pricing (Swen et al. 2007, p. 3). On the patient scale, I would argue that by removing the guesswork of determining which drugs would work best for individual patients; pharmacogenomics will actually reduce the amount of money patients spend on drug treatments, as they would not have to pay for ineffective drugs or treatment for severe negative side effects.
One more argument that educating the public on pharmacogenomics and genetic analysis of their diseases is that it would be a waste of time and effort, as ordinary people are not interested in these subjects. However, I would disagree. Research has shown that the more educated people are on pharmacogenomics and its actual benefits and drawbacks, the more supportive they are towards implementing pharmacogenomics into clinical settings are a larger scale (Nielsen and Møldrip 2006, p. 5). I believe that once people realize how important pharmacogenomics is to protecting and improving their health, they will not only demand that there be more resources dedicated to researching pharmacogenomics, they will also place pressure on lawmakers to develop regulations for it so that personalized medicine can be applied to clinical settings. Through greater public understanding and support, pharmacogenomics can overcome its limitations and achieve greater presence in the clinic.
VI. Conclusion
Pharmacogenomics has already made great contributions to improve drug therapies by making treatment more targeted and efficient, and it has the potential to improve them even further. Researchers have identified hundreds of known interactions between gene mutations and drug responses, and as genetic analysis technology and methods improve, the future holds more discoveries. Pharmacogenomics is also crucial to improving drug safety, as genetic analysis predicts whether a patient will experience ADRs from a specific drug before prescribing the drug. However, in order for pharmacogenomics to be truly effective and accessible, it must address and overcome several challenges, such as cost, lack of research, regulations, privacy concerns, and lack of education among doctors and the public. Fortunately, for the future of pharmacogenomics, there are solutions to these challenges. As technology for genetic analysis advances, the price of genetic sequencing and pharmacogenomics falls. With recent discoveries and growing interest in our personal genomes, scientists explore this new and potential-filled field conducting new research. Additionally, the education of doctors and the public on pharmacogenomics will be crucial in helping personalized medicine become more widespread. When people understand what pharmacogenomics is and how it can protect their own health, they will be more open to the use of personalized medicine in the clinical setting. As more research and greater public interest in pharmacogenomics accumulates, it will become easier for pharmacogenomics and use of personalized medicine to enter the mainstream medical toolkit.
Despite the obstacles preventing pharmacogenomics from being more widespread, its potential benefits are worth further research and analysis in the field. Already researchers are investigating new possible gene and drug reactions for several different diseases, including cancer, heart disease, and depression, and coming up with innovative ways to study and test pharmacogenomics. There has also been a greater push recently to educate the public on pharmacogenomics for easier implementation. Though the challenges are daunting, they are surmountable, and the benefits that pharmacogenomics would bring to a wider group of patients outweighs the costs of implementing and distributing personalized medicine.
I hope that by presenting my research, I can spark greater interest for pharmacogenomics in many people, and encourage more research and support for the field. If my project inspires more students to research pharmacogenomics, then there would be more scientists to study all of the different genes and drugs of pharmacogenomic interest. I also hope that raising awareness of pharmacogenomics will lead to greater popular support and a push for common usage of genetic analysis clinical settings. With this increased support and funding, researchers could investigate and find new ways to improve the effectiveness and accessibility of pharmacogenomics, providing better drug treatments and better health for everyone. Pharmacogenomics is truly a revolutionary field with great potential, and it deserves to be more widespread in clinical settings so that more people can take advantage of its benefits. However, that can only happen if we dedicate the effort and resources to better understand and implement pharmacogenomics, so that it can overcome the obstacles in its way and provide better medical treatments for everyone.
Sources
Center for Education and Research on Therapeutics (CERT) at Georgetown University. Preventable Adverse Drug Reactions: A Focus on Drug Interactions. Silver Spring (MD): U.S. Food and Drug Administration; [2018 Mar 6, accessed 2018 Apr 9] Available from: https://www.fda.gov/Drugs/DevelopmentApprovalProcess.
ClinicalTrials.gov. Feb 2000 – Dec 2017. Bethesda (MD): Nat Library Med at NIH; [accessed 2018 Jan 17]. Available from: https://clinicaltrials.gov/search/term=pharmacogenomics+OR+pharmacogenetics.
Fact Sheet 21: Pharmacogenetics/Pharmacogenomics. New South Wales (Australia): Centre for Genetics Education; [2015 Sept 30; accessed 2018 Jan 17]. Available from: http://www.genetics.edu.au/publications-and-resources/facts-sheets/fact-sheet-21-pharmacogenomics-pharmacogenetics.
Frequently asked questions about pharmacogenomics. 2016 May 2. Bethesda (MD): National Human Genome Research Institute; [2016 May 2; accessed 2018 Jan 17]. Available from: https://www.genome.gov/27530645/.
Gardiner SJ, Begg EJ. 2006. Pharmacogenetics, drug-metabolizing enzymes, and clinical practice. Pharmacological Reviews. [2006 Sept; accessed 2018 Jan 24]; 58(3):521-590. Available from: https://doi.org/10.1124/pr.58.3.6.
Hamburg M, Collins F. 2010. The path to personalized medicine. N Engl J Med. [2010 Sept 8; accessed 2018 Jan 17]; 363(4):301-304. Available from: http://www.nejm.org/doi/full/10.1056/nejmp1006304#t=article
Higashi MK, Veenstra DL, Kondo LM, Wittkowsky AK, Srinouanprachanh SL, Farin FM, Rettie AE. 2002. Association between CYP2C9 genetic variants and anticoagulation-related outcomes during warfarin therapy. JAMA. [2002 Apr 3; accessed 2018 Jan 24]; 287(13):1690–1698. DOI:10.1001/jama.287.13.1690.
Katsios C, Roukos D. 2010. Individual genomes and personalized medicine: Life diversity and complexity. Personalized Medicine. [2010 Jul 5; accessed 2018 Jan 17]; 7(4): 347-350. Available from: https://www.futuremedicine.com/doi/full/10.2217/pme.10.30.
Kimmel SE, French B, Kasner SE, et al. 2013. A pharmacogenetic versus a clinical algorithm for warfarin dosing. N Engl J Med. [2013 Dec 12; accessed 2018 Jan 24]; 369:2283-2293. DOI: 10.1056/NEJMoa1310669.
Kitzmiller JP, Groen DK, Phelps MA, Sadee W. 2011. Pharmacogenomic testing: Relevance in medical practice. Cleve Clin J Med. [2011 Apr; accessed 2018 Jan 17]; 78(4):243–257. Available from: https://www.ncbi.nlm.nih.gov/pmc/articles/PMC3351041/.
Ko TM, Wong CS, Wu JY, Chen YT. 2016. Pharmacogenomics for personalized pain medicine. Acta Anaesthesiologica Taiwanica. [2016 Mar; accessed 2018 Feb 26]; 54(1):24-30. Available from: https://doi.org/10.1016/j.aat.2016.02.001
McLeod HL. 2013. Cancer pharmacogenomics: Early promise, but concerted effort needed. Science. [2013 Mar 29; accessed 2018 Jan 31]; 339(6127):1563–1566. DOI:10.1126/science.123413.
Motsinger-Reif AA, Jorgenson E, Relling MV, Kroetz DL, Weinshilboum R, Cox N., Roden DM. 2013. Genome-wide association studies in pharmacogenomics: Successes and lessons. Pharmacogenet Genomics. [2013 Aug 1; accessed 2018 Jan 17]; 23(8):383–394. Available from: https://www.ncbi.nlm.nih.gov/pmc/articles/PMC3003940/.
Nielsen LF, Møldrip C. 2006. Lay perspective on pharmacogenomics: a literature review. Future Med Ltd. [2006 Aug 7; accessed 2018 Mar 25]; 3(3):311–316, Available from: https://doi.org/10.2217/17410541.3.3.311.
PharmGKB. 2001-2018. Stanford (CA): Stanford University; [accessed 2018 Jan 17]. Available from: https://www.pharmgkb.org/.
Sauna ZE, Kimchi-Sarfaty C, Ambudkar SV, Gottesman MM. 2007. Silent polymorphisms speak: How they affect pharmacogenomics and the treatment of cancer. AACR Publications. [2007 Jul 10; accessed 2018 Jan 17]; 67(20):9609-9612. Available from: http://cancerres.aacrjournals.org/content/67/20/9609.short.
Soto R, Gainford A, Stanger G. 2012. The Role of Pharmacogenetics/Pharmacogenomics in Drug Development and Regulatory Review. Jacksonville (FL). SlideShare. [2015 Aug 23, accessed 2018 Apr 4] . Available from: https://www.slideshare.net/rsoto21/pharmacogenetics-and-pharmacogenomics.
Swen JJ, Huizinga TW, Gelderblom H, de Vries EGE, Assendelft WJJ, Kirchheiner J, Guchelaar HJ. 2007. Translating pharmacogenomics: Challenges on the road to the clinic. PLoS Med. [2007 Aug 14; accessed 2018 Jan 24]; 4(8):e209. Available from: https://doi.org/10.1371/journal.pmed.0040209.
Tollman P, Guy P, Altshuler J, Flanagan A, Steiner M. 2001. A revolution in R&D: How genomics and genetics are transforming the biopharmaceutical industry. Boston(MA):Boston Consulting Group. [2001 Nov 1, accessed 2018 Mar 7]; Available from: https://www.bcg.com/documents/file13745.pdf
Wolfgang S, Zunyan D. 2005. Pharmacogenetics/genomics and personalized medicine. Oxford Academic. [2005 Oct 15; accessed 2018 Jan 24]; 14(2) R207–R214. Available from: https://doi.org/10.1093/hmg/ddi261