Murky Waters: Turbidity’s Effect on Aquatic Predation
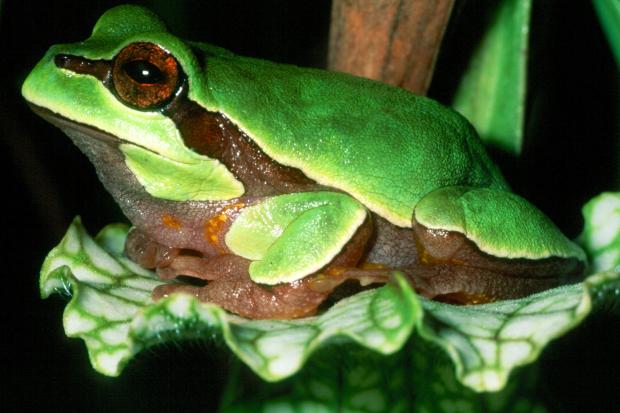
Abstract
Temporary pond ecosystems are a fascinating and underexplored kind of ecosystem. This paper aims to investigate predation within these systems, particularly in respect to temporary ponds in North Carolina and the key creatures that inhabit them. Two such creatures are the Pine Barrens tree frog and the red spotted newt. Their predatory relationship is defined by variables related to the temporary pond environment, such as turbity and the benthic zone. This paper demonstrates that increased turbidity has been shown to decrease consumptive and non-consumptive effects and trade-offs. Moreover, through the exploration of turbity and predation, this paper illustrates that temporary pond ecosystems are understudied and ripe with potential knowledge.
Introduction
If you have ever taken a walk after a thunderstorm, you might notice a few puddles that have formed as a result of the rain. These puddles represent a potentially important ecosystem that, comparatively speaking, we know almost nothing about: temporary pond ecosystems. Temporary pond ecosystems are bodies of water created by both rainfall and runoff, which are completely dry for part of the year (Pinto-Cruz et al., 2011). This drying out process creates a unique aquatic habitat where there are no fish in the ecosystem, as they are unable to cope without water (Wilbur, 1997). Instead, amphibians, such as the Pine Barrens tree frog, a near threatened species, and the red spotted newt, a keystone species, dominate the food web. These creatures make temporary pond ecosystems ecologically important (Wilbur, 1997). This change in speciation allows for interesting predator-prey interactions that have associated effects, known as consumptive and nonconsumptive effects, of predation.
There are two categories of the effects of predator-prey interactions: lethal and non-lethal. Lethal or consumptive effects (CEs) involve the predator killing the prey, reducing prey population (Lima, 1998). Non-lethal or nonconsumptive effects (NCEs), also known as antipredator behavior, are behavioral shifts in the prey population, including a change in habitat and activity level (Lawler, 1989; Lima, 1998). Decisions surrounding NCEs are often made through a tradeoff analysis by each individual animal, similar to cost-benefit analyses in economics (Lima, 1998). Some studies have looked at turbidity’s effects on fish predator-prey interactions, while others have examined how various aspects of NCEs change in predator-prey relationships between Pine Barrens tree frogs and red spotted newts when other variables are manipulated (Abrahams & Kattenfeld 1997; Lawler, 1989). However, nothing currently exists detailing how turbidity, which is the amount of particles suspended in water, changes the NCEs of the relationship between Pine Barrens tree frogs and red spotted newts. To further understand the mechanisms that affect predation, I analyzed and synthesized scholarly journal articles and popular sources to determine the effect that turbidity has on the predation of Pine Barrens tree frogs by red-spotted newts in southeastern North Carolina temporary ponds.
In this paper, I show that turbidity reduces the NCEs of the interactions, specifically in regards to the activity level and location of the Pine Barrens treefrog larvae within the ecosystem. It also shows that turbidity will reduce the CEs associated with this relationship, by decreasing visibility in the water for both predator and prey, leading to fewer encounters and kills, along with a reduction of tradeoffs, due to the reduction of NCEs and CEs. This research is vital to understanding how the environment affects the organisms living in it, and how in turn the organisms affect each other, specifically in temporary pond ecosystems. It could be applied to current models of predation to make them more accurate and inclusive. Perhaps most importantly, this research adds to the current data surrounding biodiversity preservation. In order to better preserve nature’s biodiversity, we must have a more complete understanding of how the separate parts of ecosystems function. For temporary ponds, more research is necessary to further understand how they function and what their potential value is to the greater ecosystems of which they are a part. To that end, this specific research increases the knowledge surrounding this ecosystem, preparing for more effective preservation of biodiversity in southeastern North Carolina temporary pond ecosystems.
This paper will cover each aspect of my project separately, ending with a synthesis of each component. To start, this paper will review the current research and ideas on the specific aspects of predation. It will look at each species previously mentioned, take a more in-depth examination of temporary pond ecosystems, and look at the differences between permanent and temporary pond ecosystems with emphasis on food web structure and speciation. Then, the consumptive and nonconsumptive effects of predation will be re-examined, focusing on the dependent variables of microhabitat shifts and activity level that I researched, followed by a short discussion of tradeoffs. I will then discuss the independent variable, turbidity level. Finally I will examine how all these variables interact and combine into a the single coherent idea. I will then conclude and reiterate the importance of my research, with the end goal to increase the knowledge surrounding southeastern North Carolina temporary pond ecosystems.
Literature Review
In the field of behavioral ecology, predator-prey interactions are often discussed when assessing why animals interact with each other the way they do. Currently, there is consensus among experts that predation has both consumptive and nonconsumptive effects (Lima, 1998). According to Lima (1998), to have a more complete understanding of how predation functions, consumptive and nonconsumptive effects (CEs and NCEs) must be considered equally. Also, when examining nonconsumptive effects and prey decision-making, tradeoffs must be taken into account (Lima, 1998). In tandem with the effects of predation, another trend in behavioral ecology studies how these effects change in response to external environmental factors, such as turbidity. Understanding how all of these pieces fit together aids us in adding to the knowledge surrounding this subject and in preserving biodiversity.
Consumptive effects of predation are best summed up with a simple expression: predator eats prey. This leads to a decrease in the prey population and an increase in the predator population (Stevens, 2012). In an ideal system, an increase in predators and decrease in prey leads to a higher predator to prey ratio than is sustainable, causing a decrease in the predator population and an increase in the prey population; the cyclical pattern is known to us as the Lotka-Volterra model, as detailed by Stevens (2012). However, predation is rarely so simple and cyclical; these variations can be caused by a myriad of factors, including multi-predator or multi-prey systems and limitations of food for the prey (Stevens, 2012). Another possible variable in the not-so-cyclical nature of predation is the idea of nonconsumptive effects of predation, or antipredator behavior.
Antipredator behavior comes in several different forms, including foraging for food, microhabitat shifts, camouflage, changes in activity level, and emigration of a habitat (Abrahams & Kattenfeld 1997; Lawler, 1989; Lima, 1998; Rosier, 2011). For H. andersonii larvae, Lawler found that they became more benthic (i.e. they resided at the lower levels of the pond) and had lowered activity levels in response to N. v. viridescens. They even became immobile at stages (Lawler, 1989). This exhibited behavior was in stark contrast to normal conditions, where they were seen to bob occasionally, a behavior not found in other species (Lawler, 1989). Antipredator behavior has certain costs associated with it. Without these costs, antipredator behavior would simply be the normal behavior of prey (Abrahams & Kattenfeld, 1997). These costs include a decrease in energy intake, decreased Darwinian fitness, possible reduced growth rates, and microhabitat shifts to less productive habitats (Lima, 1998; Nelson et al., 2004). However, there are benefits that result from antipredator behavior, including a reduction in predator detection that leads to a higher chance of survival over the long run (Lima, 1998). All of these studies show that antipredator behavior has both costs and benefits associated with it, which much be assessed on an individual basis. This decision-making process can be understood in terms of tradeoffs.
Tradeoffs are essential to understanding the behavior in behavioral ecology, especially concerning predator-prey interactions (Lima, 1998). Prey are faced with a decision about whether to risk being eaten for energy intake, or reduce risk taking, leading to lower mortality (Lima, 1998). Lawler draws some interesting conclusions in her experiment with H. andersonii larvae, which highlights the essential nature of tradeoffs. Her findings suggest that predation selects for a reduction in prey activity level, while the drying out process in temporary ponds selects for normal to heightened activity levels to increase growth (Lawler, 1989). In this type of scenario, there should exist an optimal tradeoff between the costs and benefits of antipredator decision making; however, attaining such an optimal state can prove difficult (Lima, 1998). In a more recent study done by Dr. Orrock et al. (2013) at the University of Wisconsin, the tradeoff that aquatic animals make between taking refuge or immediately becoming prey can have long-lasting negative effects, including growth and fecundity. This conclusion is upheld by studies done at University of California-Davis, where Nelson et al. (2004) found that pea aphids had reduced growth rates as a result of increased antipredator behavior. These various results show that the tradeoff decision is important to animals across various taxa, as their survival and Darwinian fitness depend on what they decide. This decision is altered, as are antipredator behavior and the consumption rate, in response to turbidity.
Turbidity in aquatic predator-prey interactions has been found to have significant effects on both consumptive and nonconsumptive effects of predation (Abrahams & Kattenfeld, 1997). In his study, Abrahams (1997) found that turbidity decreased the antipredator behavior exhibited by the prey, fathead minnows. He also found that turbidity increased the consumptive effects between fathead minnows and yellow perch (Abrahams & Kattenfeld, 1997). In another experiment, Chivers et al. (2013) found that turbidity impaired fathead minnows’ ability to distinguish predators from non-predators, leading to an overall decrease in antipredator behavior. In addition, an experiment run at the University of Helsinki in 2013 showed that turbidity around 30 NTUs increased the consumptive effects of predation, due to a decrease in antipredator behavior between Perca fluviatilis and its prey, plankton (Pekcan-Hekim et al., 2013). All of these experiments point towards an increase in consumptive effects, in part due to a decrease in antipredator behavior, as a result of increased turbidity.
All of the research currently available on this topic indicates that both consumptive effects and antipredator behavior are necessary to understanding predator-prey interactions. In addition, the decision-making process associated with antipredator behavior is done through an analysis of the costs and benefits of the decision by the individual, known as tradeoffs. These effects in aquatic environments are altered when turbidity enters the ecosystem, lowering antipredator behavior and increasing consumptive effects. These results support my hypothesis in part; however, the idea that consumptive effects of predation will increase due to turbidity does not agree with my hypothesis. The difference from what is considered conventional is accounted for in my own study. This review brings together studies from various systems to gain a better understanding of what the consensus amongst the academic community is concerning the different sides predation, with a concentration on aquatic systems. Now, what follows is closer look at the associated species, the habitat where the interactions take place, and how the effects of predation change in response to an increase in turbidity.
Independent Research
In order to understand how the predator-prey relationship between Pine Barrens tree frogs and red-spotted newts functions, we need to examine the species at hand, starting with the prey, Hyla andersonii, also known as the Pine Barrens tree frog. This species ranges from New Jersey to Louisiana, going down the Atlantic Coast and across the Gulf of Mexico (New Jersey, 2014). In North Carolina, they are known to be found specifically in the Sandhills region, along with the greater southeastern section of the state (Wilbur, 1997). They are also considered a near threatened species, making them of greater ecological importance. When discussing this predator-prey relationship, we are concerned with the larval stage of the animal. This stage is also known as the tadpole stage, occurring between hatching and the completion of metamorphosis. Females lay their eggs singly, as opposed to a clump method, in aquatic environments, often at the bottom of ponds (New Jersey, 2014). When they hatch, tadpoles emerge, grow, and mature within the habitat they are born (New Jersey, 2014). As adults, they average between 1.5-2 inches in length, or 38-51 mm (New Jersey, 2014), which tells us the tadpoles are quite small. They have average activity levels within their habitat; they swim and move around readily (Lawler, 1989). They also normally live in the higher regions of the pond ecosystem, not in the benthic or bottom layer (Lawler, 1989). However, these characteristics change in the presence of a common predator, the red-spotted newt (Lawler, 1989).
The red-spotted newt, or Notophthalmus viridescens viridescens, is one of four subspecies of the eastern newt, N. viridescens (Riemland, 2000). For this animal, we are interested in the adult stage. The red-spotted newt is approximately four to five inches in length, and is found in similar areas as the Pine Barrens tree frog (Riemland, 2000; Wilbur, 1997). The adults spend the majority of their time in small bodies of freshwater, and have been found in temporary pond ecosystems in southeastern North Carolina (Wilbur, 1997). If necessary, adults can live on land, especially when the water level runs low (Riemland, 2000). They have also been known to bury themselves into mud or dirt underground during the winter to outlast the cold and wait for the return of the rain (Riemland, 2000). They have a varied diet, ranging from insect eggs to wood frog tadpoles, hence the relationship between the two species (Gill, 1978; Riemland, 2000). Along with their status as predators within temporary ponds, they have also been recognized as a keystone species within southeastern North Carolina temporary ponds, giving them even greater ecological importance within the ecosystem (Wilbur, 1997). A keystone species is one who is crucial for maintaining ecosystem structure and function; its removal changes the ecosystem significantly (Wagner, 2012). As such, understanding how this top predator interacts with its prey will make it easier to understand how the food web and ecosystem function. Speaking of ecosystems, where do these interactions take place?
Temporary pond ecosystems are vastly different from regular ponds. Previously mentioned were some of the starker differences between temporary and permanent ponds, including the drying out process and the lack of fish. However, the importance of this lack of fish may have been understated. This allows for domination of the ecosystem by amphibians, providing them with an important breeding habitat (New Jersey, 2014; Riemland, 2000, Wilbur, 1997). While the speciation, or types of species inhabiting the ecosystem, may be different, temporary ponds are just as complex (Collinson et al., 1995; Schneider & Frost, 1996). In particular, the macroinvertebrate communities, part of the base of the food web of temporary pond ecosystems, were found to be just as complex, even with the drying out process (Collinson et al., 1995). As such, predator-prey interactions are common in the ecosystem. Next, consumptive effects, nonconsumptive effects, and tradeoffs are determined to be a variable in the case of Pine Barrens treefrog larvae and red-spotted newts without turbidity.
When considering the relationship between Pine Barrens treefrog larvae and red-spotted newts, the consumptive effects are quite simple: the newts eat the larvae. As mentioned earlier in the literature review, this decreases larval population and increases newt population. In addition to that, as previously mentioned, larvae exhibit antipredator behavior under normal conditions in the form of a reduction in activity level and a more benthic microhabitat (Lawler, 1989). They are also forced to make a decision about when to change their behavior, driven by a desire not to be eaten and countered with a need for food and growth, so as to outlast the pond’s drying out process and reach maturity, increasing Darwinian fitness (Lawler, 1989; Lima, 1998). (For more information, one should refer to the literature review for a more complete explanation of consumptive effects, nonconsumptive effects, and tradeoffs). Before we put this all together, one more aspect must be looked at: the independent variable, turbidity.
Turbidity, defined earlier as the amount of particles suspended within a body of water, is created through several mechanisms, including phytoplankton within the ecosystem, anthropogenic pollution, and algae that can be increased by runoff and anthropogenic pollution. Turbidity affects our specific ecosystem by lowering the amount of light reaching the bottom of the pond and by decreasing visibility for the concerned species (Abrahams & Kattenfeld, 1997). Now, how do all of these pieces fit together?
Discussion
Starting with turbidity’s effect on CEs, my research shows that an overall decrease in CEs will occur as a result of increased turbidity. This is because turbidity decreases the visibility within the ecosystem for both predator and prey, leading to fewer predator-prey encounters. I also think that the varied newt diet and habitat shifts allows for high flexibility in what it eats (Riemland, 2000). Therefore, if it becomes too much of a burden for the newt to try and eat the tadpoles, then it will simply switch to another prey species and attack that. This would lead to a decrease in the CEs of the newt-tadpole relationship. While Abrahams (1997) found that turbidity would increase the lethality of aquatic predation, his experiment was run using fish species as the predator and prey. This is different than mine, as newts have the ability to switch to other food sources outside the water if necessary. Also, Abrahams (1997) did not allow the predator to have another food option, meaning that they had to wait for the prey, rather than moving on to other food sources, as the newts could do. So, in the case of Pine Barrens treefrog larvae and red-spotted newts, turbidity decreases CEs.
In addition to a reduction of CEs, my research also shows that NCEs are lowered when turbidity is increased within the ecosystem. This is due to both decreased visibility and lower amounts of light reaching the benthic regions (Abrahams & Kattenfeld, 1997). As previously mentioned, under normal circumstances the tadpoles become more benthic and reduce their activity in the presence of predators. However, with decreased visibility, detection of predators becomes more difficult, leading to less antipredator behavior and normal activity levels, which is consistent with Abrahams’ (1997) findings, among various other scholars referenced in the literature review. There also will not be a need for the tadpoles to shift to the benthic zones of the pond. In regards to the benthic zone, the lower amount of sunlight reaching that region leads to lower photosynthesis production, reducing the amount of food and species there. Regardless of visibility levels, tadpoles are probably more prone to risk of an encounter with a predator when staying higher in the pond. Nevertheless, they do this in order to feed and increase Darwinian fitness. This is a classic tradeoff situation. Speaking of tradeoffs, how does turbidity affect those? Well, with a decrease in both CEs and NCEs, meaning increased energy input along with an increased survival rate, much less is risked for more gain than normal circumstances. This leads to a decrease in tradeoffs. The decision is simple: stay high in the ecosystem and feed to increase Darwinian fitness. In short, increased turbidity leads to a decrease in CEs, NCEs, and tradeoffs associated with the predator-prey relationship between red-spotted newts and Pine Barrens treefrog larvae. So, why is this important?
Conclusion
Currently, we do not know enough about predator-prey interactions in temporary pond ecosystems, or even about the ecosystems themselves. This research helps to fill a gap in our understanding of how turbidity affects both the predator-prey relationship between Pine Barrens tree frogs and red spotted newts, and how it affects the greater food web and ecosystem structure. It gives us precious knowledge regarding a keystone species, which helps us better understand how the food web functions, and to an extent how the ecosystem functions (Wilbur, 1997). This will help us better preserve its biodiversity, since an increase in scientific knowledge helps policy makers create more effective habitat and species conservation policies. However, more research must be done surrounding this issue. In regards to my specific question, an actual experiment measuring the effects of turbidity on predation between the species would go a long way in augmenting the conclusions I have drawn here. Other questions about turbidity should also be studied. For example, at what level of increased turbidity do these effects begin to take place? Or how does the amount of other prey for the newt change the effects of the relationship between the newts and the larvae? Research can be expanded to several other issues surrounding this ecosystem. For example, how does increased turbidity affect not just these two species, but the greater ecosystem structure? Can these same effects be observed under different environmental stimuli, such as increased aquatic vegetation, or will they be altered? What policy changes must be effected in order to maintain these ecosystems? Many unanswered questions surround this ecosystem, which needs to change. We must preserve these ecosystems in order to fulfill our role as stewards over our planet by preserving and maintaining crucial biodiversity.
Sources
Abrahams M, Kattenfeld M. 1997. The role of turbidity as a constraint on predator-prey interactions in aquatic environments. Behav Ecol Sociobio. 60:169-174.
Chiver DP, Al-Batati F, Brown GE, Ferrari MCO. 2013. The effect of turbidity on recognition and generalization of predators and non-predators in aquatic ecosystems. Ecol Evol. 3(2): 268–277.
Collinson NH, Biggs J, Corfield A, Hodson MJ, Walker D, Whitfield M, Williams PJ. 1995. Temporary and permanent ponds: an assessment of the effects of drying out on the conservation value of aquatic macroinvertebrate communities. Biol Conserv. 74:125-133.
Fabian D, Flatt T. 2011. The evolution of aging. Nature Education Knowledge 3(10):9
Gill DE. 1978. The metapopulation ecology of the red-spotted newt, notophthalmus viridescens (rafinesque). Ecol Monographs. 48:145-166.
Lawler SP. 1989. Behavioural responses to predators and predation risk in four species of larval anurans. Anim Behav. 38:1039-1047.
Lima SL. 1998. Non-lethal effects in the ecology of predator-prey interactions. Bioscience 48(1):25-34.
Nelson EH, Matthews CE, Rosenheim JA. 2004. Predators reduce prey population growth by inducing changes in prey behavior. Ecology. 85(7):1853–1858.
New Jersey endangered and threatened species field guide: Pine Barrens tree frog [Internet]. Trenton (NJ): Conserve wildlife foundation of New Jersey; [cited 2014 Mar 17]. Available from: http://www.conservewildlifenj.org/species/fieldguide/view/Hyla%20andersonii/
Orrock JL, Preisser EL, Grabowski JH, Trussel GC. 2013. The cost of safety: Refuges increase the impact of predation risk in aquatic systems. Ecology. 94(3):573-579.
Pekcan-Hekim Z, Joensuu L, Horppila J. 2013. Predation by a visual planktivore perch (Perca fluviatilis) in a turbulent and turbid environment. Can J Fish Aquat Sci. 70(6): 854-859.
Pinto-Cruz C, Barbosa AM, Molina JA, Espírito-Santo MD. 2011. Biotic and abiotic parameters that distinguish types of temporary ponds in a Portuguese Mediterranean ecosystem. Ecol Indic. 11:1658-1663.
Riemland, S: Notophthalmus viridescens [Internet]. Ann Arbor (MI): Animal Diversity Web; [updated 2000, cited 2014 Mar 17]. Available from: http://animaldiversity.ummz.umich.edu/accounts/Notophthalmus_viridescens/
Rosier RL, Langkilde T. 2011. Behavior under risk: How animals avoid becoming dinner. Nature Education Knowledge. 2(11):8-11.
Schneider DW, Frost TM. 1996. Habitat duration and community structure in temporary ponds. J N Am Benthol Soc. 15(1):64-86
Stevens A. 2012. Dynamics of predation. Nature Education Knowledge. 3(10):46-48.
Wagner SC. 2012. Keystone species. Nature Education Knowledge. 3(10):51-53.
Wilbur HM. 1997. Experimental ecology of food webs: complex systems in temporary ponds. Ecology. 78(8):2279-2302.