Regenerative Medicine: The Interplay of Stem Cells and Polymer Science
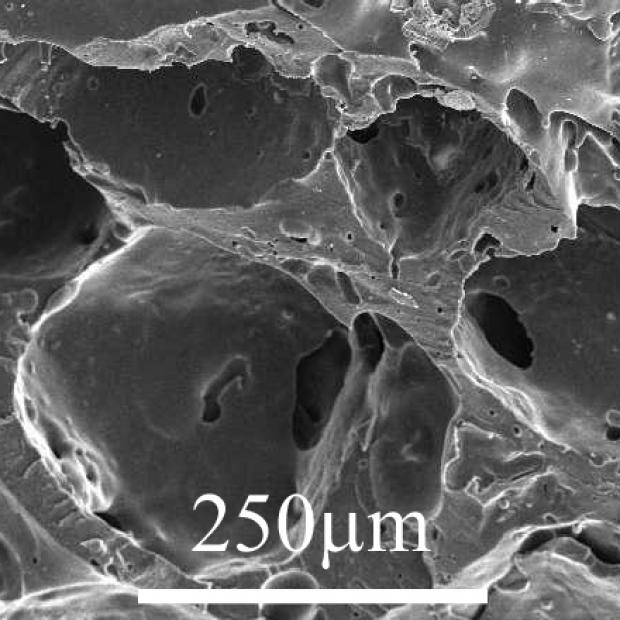
Abstract
Regenerative medicine is one of the most exciting frontiers of medicine. It has the potential to save millions of lives through the growth of new, healthy organs. People die every day waiting on organ transplant lists that might soon be unnecessary. This paper looks at early progress made in the field, such as Harvard’s Dr. Operpenning, who grew a new bladder using early scaffolding techniques, and then takes us through the field’s evolution. Scientists can now use 3D printing methods to create biodegradable scaffolds that can be placed into a patient’s body to help them grow a new organ. Researchers will soon be able to create any organ, from kidneys to bladders to sex organs. But scaffolds require cells to function inside a human body. Stem cells in particular are required to make this revolutionary technique work, and the political rhetoric surrounding stem cells has created a barrier that has crippled the progress of regenerative medicine. Another innovation this paper explores is the decellularization process, by which a donor organ has the outer cells removed from its tissue structure. The residual structure can then be coated in the patient’s cells. This method widely increases the donor base for organ transplants. Regenerative medicine not only has the potential to save lives; it also synthesizes disparate fields, pairing tissue engineers with surgeons, pharmacists, and a wide array of scientists. This combination of expertise is the gold standard for scientific cooperation.
On the first night of my sophomore year of high school, I woke up in the worst pain I had ever felt. I thought I was dying. The unfortunate reality was that I was not entirely wrong. I would have died that night had it not been for the skill of the surgeons at Moses Cone Hospital in Greensboro. I learned that I carried a birth defect known as Meckel’s Diverticulum. This is a condition which affects about 2% of the population, and it involves an abnormal growth on the small intestine. In most cases, this growth does no harm, but in a small number of people, including myself, the growth ruptures and the situation becomes more serious. The care I received during this experience not only fueled my interest in medicine, but also led me to reflect on birth defects in general, particularly those relating to vital organs.
The leaps and bounds that surgical methods and technology have taken towards improvement have made it possible for people with relatively minor defects to survive. Procedures that were in the realm of science fiction merely a few decades ago are now the norm. The idea that birth defects and organ failure can generally be cured by transplants and tissue repairs is increasingly taken for granted. However, due to the large number of people in need of transplants, and the shortage of donor organs available, we have created a faulty system of endless waiting lists and deadly complications. With our population rapidly aging, the number of patients needing a transplant has doubled, but the number of transplants taking place has remained stagnant (Atala, 2009). The solution to this problem lies closer than one might realize.
Growing organs might seem like a futuristic endeavor, but the reality is that successful experiments have been conducted since the 1990s and that it is one of the most rapidly expanding fields in modern medical science. What started as regenerating tissues in camp bladders has turned into using 3D-printing methods to engineer biodegradable organ scaffolds which are inserted into the body, allowing for the growth of a new organ (Campodonico et al., 2004). This has been successfully achieved with bladder transplants in people born with a defect which does not allow their bladders to expand properly – leading to the impairment of their kidneys and the need for dialysis. Currently a person dies every 30 seconds by something which could have been prevented by the regeneration of organs (Atala, 2009). This part of medical science is collectively known as regenerative medicine, and I sincerely believe it is the most important research being conducted right now. It is a vast and complicated field, highlighting the interdisciplinary nature of modern pragmatic science, particularly between the surgical and polymer-chemistry units.
Reconstruction methods for the urinary bladder in canine models were developed in an early study of modern regenerative techniques. This feat was performed by Dr. Oberpenning’s lab at Harvard Medical School in 1999. This was the first study to ever prove that autonomous reconstruction of hollow organs is possible, something which was achieved by creating a so called “neo-bladder” from cells harvested from the subjects native bladder (Oberpenning et al., 1999). The collected cells were grown in cell-cultures to produce urothelial and smooth muscle cells to be spread across a bladder-like polymer structure. This structure was then transplanted into the subject, and function was evaluated over a period of eleven months. At the end of this period every value was found to be within normal conditions (Oberpenning et al., 1999). This study is crucial in understanding the beginnings of regenerative discovery. The third author of the paper presenting this particular case is Dr. Anthony Atala, current director of the Wake Forest Institute of Regenerative Medicine. He has played a key role in furthering the field, and this was, in addition to being the first successful neo-organ transplant, his first major publication on the topic. Atala then went on to form his own research team at Harvard, and in 2006, the paper “Tissue-engineered autologous bladders for patients needing cystoplasty” was published. Here the previous study evolved from neo-bladder implantation in dogs to implantation in human patients (Atala et al., 2006). The study involved seven children and young adults ranging from four to nineteen years of age who all suffered from a condition where their bladders were not operating properly. The previous method used to treat these bladder-related problems was cystoplasty, which has a high risk of causing severe complications, and therefore this experimental method was deemed appropriate for testing (Atala et al., 2006). Results were determined within 22 to 61 months through check-ups and bladder biopsies. Results were overall positive, and thus transplantation of engineered bladders was deemed a viable alternative to the dangerous cystoplasty procedure (Atala et al., 2006). Advancements have been relatively swift, with human trials starting so shortly after the previous animal modeling. Since then, Atala has moved to lead a research facility at the Wake Forest School of Medicine, where research at the forefront of regenerative methods continues to progress at a steady pace.
While the aforementioned surgical methods have improved steadily throughout the early 21st century, another field which is highly crucial to this success has also been prosperous in its progress: biodegradable scaffolding, as well as polymer materials. This research started making significant progress in the year 2000 when two new scaffolding techniques were produced. Dr. Shea’s and Peters’s team from the University of Michigan discovered a method to create flexible biodegradable scaffolding material through the use of crystallized polymers and foam. The results appeared to be successful, as the constructed scaffold withstood several tests of temperature, pH, and time. Furthermore, porosity was detected at well above minimum, at around 95% in the samples which were tested (Sheridan et al., 2000). Meanwhile, at the Korea Advanced Institute of Science and Technology, similar research was being conducted. This research team, led by Dr. Yoon, also discovered a certain foaming method for the development of the scaffold structure. This research went even further. The scaffold was seeded with cells from rat-models to test its efficacy in potential future organ development. Pores were determined to be the ideal size for cell implantation, with a 95% implantation efficacy (Nam et al., 2000). Dr. Yoon’s team polymer also handled and molded into any shape due to the addition of ammonium bicarbonate salts. This is a revolutionary capability (Nam et al., 2000). After the discovery of the appropriate biodegradable materials, researchers started further exploring production methods. 3D-printing technology became of particular interest as early as 2002 when the National University of Singapore conducted an experiment involving the creation of several shapes using starch-based 3D printing. It was confirmed that these modern methods could eventually streamline the scaffolding process using the previously mentioned polymer-based substances (Lam et al., 2002). Biodegradable scaffolding is currently the most advanced process in the field. Simply put, a polymer model of an organ is printed by a 3D-printer (Lam et al., 2002). This model is then doused with live cells and transplanted into the body. The clinical trials that have been run with human subjects have worked with the bladder. The idea is that when we have a small scratch or cut our body knows how to repair the damage without complication, but when there is a deep wound the body makes errors and as a result, scar-tissue is created. The scaffold is engineered in such a way that it degrades within the body in layers, “confusing” the body into repairing the bladder in very small segments at a time. This makes for perfect replication of a bladder once the scaffold is completely degenerated.
One of these success-stories is exemplified by Luke Massella, who had a birth defect that caused urine to travel backwards into his kidneys, causing severe problems. 3D-printing technology was not useable yet, so his scaffolds had to be engineered by hand; however, the same principles that I discussed earlier applied. Current technology can already fix issues like this and the future looks even brighter. The main reason the first organ regeneration was of a bladder is because it is considered a simple structure. Essentially, the bladder is a sphere with one kind of muscle cell on the outside and one kind of protective cell on the inside. There are also few nerves and blood vessels to worry about (Atala et al., 2006).
Everything from windpipes and kidneys, to male and female genitalia, have been, or will soon be, successfully created. In the near future, scientists hope to develop better methods for modeling the vascular system, nerve-endings, and more complex muscle tissue. Most of the research in progress that I saw at Wake Forest was related to kidney regeneration, as this is the next logical step in terms of simplicity. However, I did have a chance to see petri dishes containing living, synthetically cultivated, heart cells which were beating independently inside their plastic vessels. The same astonishing observations of cells intrinsically knowing their function could be seen in engineered vascular tubing which expanded and contracted like normal arteries inside the human body.
The biodegradable scaffolds do, however, need cells to function inside the body. Embryonic stem-cell cultivation research is currently being done to obtain the required cells for the vast array of organs in need of engineering. This is a problematic and highly publicized topic with severe ethical implications. The first clinical human trial using embryonic stem-cells for regenerative purposes was recently announced. The idea is to be able to cure severe cases of macular degeneration, a fairly common ocular disease which can cause blindness, by growing retinal tissue and using this tissue to repair the patient’s vision (Tibbetts et al., 2012). Some might argue that the ethical difficulties presented by the use of embryonic stem-cells in this particular trial outweigh its potential benefits. This viewpoint is supported by the idea that these trials cannot be properly modeled with animal subjects before human testing, and are too dangerous to warrant the use of stem-cells. Another argument might be that macular degeneration is not severe enough of a condition to warrant this treatment. However, I think that a strong case can be made for why these trials should be allowed to proceed. One has to consider that the purpose of these clinical experiments is not only to potentially cure macular degeneration, but also to advance the field of regenerative medicine as a whole. Even if the disease in question is not be life-threatening, it is currently the most promising venue to start discovering stem-cell usage, and therefore it is our best option. If these experiments were performed we could learn more about how exactly stem cells could be used in various ways regarding regeneration of tissue. The only way we can fill in the gaps left by these unsatisfactory animal models is by proceeding cautiously to promising human trials. This would reduce the risk involved in future trials, and would therefore diminish the fear that risk outweighs reward. So, allowing trials such as this one is necessary to make concrete advancements in regenerating tissue. Eventually, these techniques might be applied to organ scaffolds and more complex tissue-structures.
The decellularization process is almost more impressive than the previously mentioned scaffolding method. In this process a donor organ is washed in a shower of different chemicals which completely remove the outer coating of cells from the tissue structure. This leaves a ghost-like organ structure with no functional cell structure. The structure is then coated in cells from the patient in need of transplantation. This method opens up the donor-base and streamlines the process by which patients are matched to organ donors (Youngstrom et al., 2013).
While all of this technological advancement and tangible evidence of success is astonishing, regenerative medicine is important for another reason. Some profound ideological inferences can be drawn from the field. My visit at Wake Forest’s regenerative labs made something very clear to me: there was a need for incredible collaboration in order to achieve these goals. The entire premise of regenerative medicine is based on taking an interdisciplinary approach to scientific discovery and unifying several scientific fields towards one specific goal. For example, in this case, everyone from biochemists and tissue engineers to surgeons and pharmacists are involved. I had always considered scientific discovery to be restricted to one field at a time. When one thinks about how research is presented through our system of education, it is hard not to assume that there are clear-cut boundaries between the fields of science, with very little overlap. At Wake Forest, I learned that their entire complex is built in an open manner, where there are not even physical boundaries in place between different research labs, in order to encourage scientific cooperation. Scientists from all these different fields are encouraged to talk to each other, and isolation in one field is viewed as a detriment to general scientific discovery. Regenerative medicine opened my eyes to the fact that pragmatic sciences, like medicine, are not properly interconnected. This potential interconnectivity is necessary to solve the complex issues of today.
Regenerative medicine is still a field in its infancy, with many years left to show its full potential. I strongly believe that a deeper analysis of this research will yield not only tremendous medical advancements, but also insight into the unification of different disciplines of science towards comprehensive goals. Regenerative medicine is an interdisciplinary field, with surgery and polymer chemistry standing as the two base pillars, one not being able to function without the other. It is particularly interesting to note how different the lab environments for these discoveries were, from Harvard Medical School’s urology department to the Laboratory of Biomedical Engineering at the National University of Singapore. This further reiterates the point that the field is reliant on a vast array of talents and technologies. This collaborative nature is something which should be further explored, as creating this type of meta-analysis of the field might aid in cutting both costs and time of research. Regenerative medicine has the potential to not only be one of the most advanced and swiftly growing fields in the pragmatic sciences, but also to be an example of life-saving scientific cooperation.
Sources
Tibbetts MD, Samuel MA, Chang TS, Ho AC. Stem cell therapy for retinal disease. Curr. Opin. Opthalmol. 23, 226–234 (2012).
Anthony Atala: Growing New Organs [Internet]. 2009, TEDMED; [cited 2014 Mar 18]. Available from: http://www.ted.com/talks/anthony_atala_growing_organs_engineering_tissue/
Atala A, Bauer S, Soker S, Yoo J, Retik A. 2006. Tissue-engineered autologous bladders for patients needing cystoplasty. The Lancet 367(9518):1241-1246. Available from: http://www.sciencedirect.com/science/article/pii/S0140673606684389
Campodonico F, Benelli R, Michellazi A, Ognio E, Toncini C, Maffezzini M. 2004. Bladder Cell Culture on Small Intestinal Submucosa as Bioscaffold: Experimental Study on Engineered Urothelial Grafts. European Urology 46(4):531-537. Available from: http://www.sciencedirect.com/science/article/pii/S030228380400212X
Lam C, Mo X, Teoh S. 2002. Scaffold development using 3D printing with a starch-based polymer. Materials Science and Engineering: C 20(1-2):49–56. Available from: http://www.sciencedirect.com/science/article/pii/S0928493102000127
Levenberg S, Huang N, Lavik E. 2003. Differentiation of human embryonic stem cells on three-dimensional polymer scaffolds. PNAS 100(22):12741–12746. Available from: http://www.pnas.org/content/100/22/12741.short
Mimeault M, Hauke R, Batra SK. 2007. Stem Cells: A Revolution in Therapeutics—Recent Advances in Stem Cell Biology and Their Therapeutic Applications in Regenerative Medicine and Cancer Therapies. Clinical Pharmacology & Therapeutics 82:252–264. Available from: http://www.nature.com/clpt/journal/v82/n3/full/6100301a.html
Nam Y, Yoon J, Gwan T. 2000. A novel fabrication method of macroporous biodegradable polymer scaffolds using gas foaming salt as a porogen additive. Journal of Biomedical Materials Research 53(1):1-7. Available from: http://onlinelibrary.wiley.com/doi/10.1002/(SICI)1097-4636(2000)53:1%3C1::AID-JBM1%3E3.0.CO;2-R/full
Oberpenning F, Meng J, Yoo J, Atala A. 1999. De novo reconstitution of a functionalmammalian urinary bladder by tissueengineering. Nature Biotechnology 17:149-155. Available from: http://www.nature.com/nbt/journal/v17/n2/pdf/nbt0299_149.pdf
Sheridan MH, Shea LD, Peters MC Mooney DJ. 2000. Bioabsorbable polymer scaffolds for tissue engineering capable of sustained growth factor delivery. Journal of Controlled Release 64:(1-3):91-102. Available from: http://www.sciencedirect.com/science/article/pii/S0168365999001388
Tu D, Seth A, Gil E, Kaplan D, Mauney J, Estrada C. 2012. Evaluation of Biomaterials for Bladder Augmentation using Cystometric Analyses in Various Rodent Models. Journal of Visualized Experiments (66):3981. Available from: http://www.ncbi.nlm.nih.gov/pmc/articles/PMC3486757/
Wei G, Ma P. 2004. Structure and properties of nano-hydroxyapatite/polymer composite scaffolds for bone tissue engineering. Biomaterials 25(19):4749–4757. Available from: http://www.sciencedirect.com/science/article/pii/S0142961203011542