The Energy Source of Tomorrow: Benefits of Nuclear Fusion Power
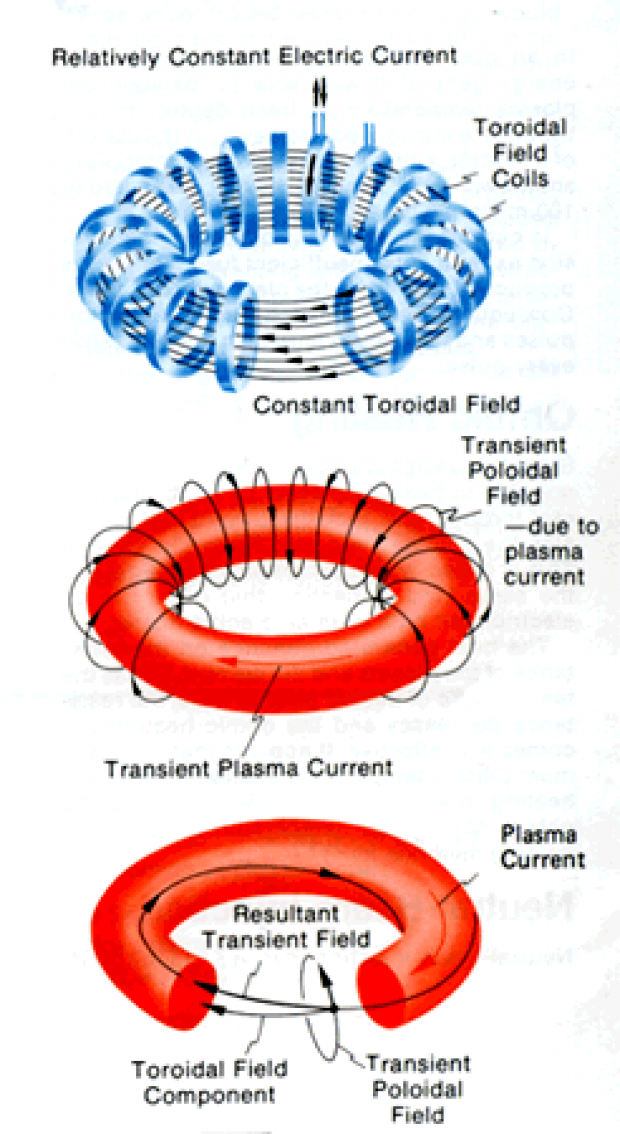
Abstract
In the search for sources of energy, discussions of nuclear fusion power as an option have often been seen as unrealistic, overshadowed by the viability of nuclear fission. Fusion power, however, would be an ideal answer to our current demand for economical and environmentally friendly energy production. This article discusses the mechanics of nuclear fusion and explains that, in terms of safety, resource availability, cost, and waste management, fusion power may be the best commercial option in the near future.
Civilization development constantly demands more efficient sources of energy, sources which would simultaneously pose minimal threat to the environment. Nuclear fusion power plants, also referred to as thermonuclear reactors, may be the best answer to the problem. Firstly, they are more efficient, and require only about one millionth of the mass of fuel needed to produce the same amount of energy as a coal operating power plant. Secondly, their fuel sources are virtually unlimited, since they are the most abundant elements in the universe. And, thirdly, they offer a much safer way of electric energy production. That is, the technologies implemented thus far are either not the safest for the environment, or not the most expedient. Thermonuclear reactors also present benefits on all three stages of processing: fuel, operation, and waste products. The quest for alternative sources of energy never stops. Even though progress in the area of fusion research has been more theoretical then practical, the field of study is constantly growing as new methods of solving problems are discovered. It is the kind of field, though, in which practice would not be possible without theory. What’s more, commercial implementation of fusion is already on the horizon. This paper will provide an overview of what particular advantages thermonuclear reactors possess, by explaining their basic principle of functioning, including fuel, operation, and waste products.
Fig.1: Basic schema of a toroidal confinement fusion reactor (tokamak)
Fuel
The theoretical idea behind the employment of nuclear fusion as an energy source is that light atomic nuclei combine to release energy. This energy comes from the difference in mass between the input material and the products of the reaction. The total mass of the reactants’ nuclei is slightly larger than the mass of produced nuclei. This excess mass is converted into energy, the amount of which can be described by Einstein’s famous rest energy equation E=mc2, which is a consequence of special relativity. In fact, this is the principal on which all currently operating nuclear power plants (using fission) are based, only in their case the mass difference arises when heavy nuclei split. As it turns out, the dividing line between the two energy releasing processes in Iron-56 (56Fe): lighter elements, which produce energy by merging and heavier ones which do so by splitting. Even though the processes are similar in nature, there is a great difference in the conditions required to facilitate both reactions.
As doctor of nuclear physics Kenneth Fowler discusses in his book, the physics of fusion is such that combination of the smallest nuclei releases the greatest amount of energy1. Thus, the most obvious fuel for a thermonuclear reaction is hydrogen and its isotopes1. Isotopes are elements which have the same number of protons but different number of neutrons in their nuclei. This property results in their identical chemical, but significantly different nuclear physical properties. There are three different isotopes of hydrogen: Hydrogen-1 (1H), common, and by far the most abundant one, Hydrogen-2 (2H), also called Deuterium (D), and Hydrogen-3 (3H), also known as Tritium (T). Out of all the combinations, the most efficient process is fusion of Deuterium (D) with Tritium (T). However, the reactions of Deuterium with itself as well as of Helium isotopes with those of Hydrogen also exhibit certain potential1. The most successful and promising design of a fusion reactor that has been developed so far is tokamak (which is a Russian acronym that stands for Toroidal Chamber with Magnetic Coils)1,2,3. It uses exactly fusion of Deuterium and Tritium and some other features because of which it is selected as an example for the following discussion. In fact, the theory behind nuclear fusion has been extensively studied and is very well developed, virtually, leaving open only the question of practical implementation.
Fig. 2: Graphic representation of a D-T reaction (2H + 3H –> 4He + 1n + 17.59 MeV Energy)
Some of the greatest benefits that fusion reactors present are fuel abundance and accessibility. Deuterium is a stable isotope and naturally occurs in place of hydrogen. In fact, it constitutes a small fraction of hydrogen in water. Quantitatively, it exists in great amounts and is virtually unlimited, taking into account how much water there is on planet Earth. According to the paper, “International Thermonuclear Experimental Reactor,” “Deuterium is really quite abundant naturally. About one part in 5000 of the hydrogen in the sea water is Deuterium. This amounts to over 1015 tons of deuterium available naturally. A single gallon of seawater would produce as much energy as 300 gallons of gasoline”4. Tritium – the second constituent of the reaction, is an unstable isotope and, for that reason, is much less abundant then Deuterium and quite rarely occurs naturally. However, this problem can be solved by a reactor design that produces Tritium during the reaction, and such design does exist. The nuclear reaction of Deuterium with Tritium produces a neutron. If the reaction space is confined inside a lithium blanket, the neutrons produced in the primary reaction will engage a secondary reaction with lithium, producing Tritium1,2,4,5. The lithium supply is also virtually unlimited, since lithium is the third most abundant element in the universe after hydrogen and helium1.
As reported by The Institute of Physics, “The long-term fuel security of fusion would appear to exceed that of fission power and hence far exceed that of fossil-fuel energy. A fusion station would use about 100 kg of deuterium and 3 tons of lithium to produce the same amount of energy as a coal-fuelled power using 3 million tons of fuel2.” Another benefit of fusion fuel is that it is quite easy to extract from raw materials. Deuterium can be separated from oxygen in water by electrolysis1,2. As a matter of fact, as a water constituent, Deuterium has a much higher density than in the separated from water gaseous state, which creates an efficient way to store it. Most of the Tritium supply is going to be produced during the reaction, and therefore no advanced storage techniques should be required. The cost price of the reactants is quite low too, and most of the expenses should be associated with building fusion power plants rather than operating them, which is the other way around for any of the current energy providing facilities2. Taking all the listed facts into account, fusion power plants have quite an advantage over any currently operating power plant type.
Another positive aspect of the fusion reactor’s fuel is that it is not harmful for the environment and safety risks associated with its storage and handling are minimal. The most important component of the reaction, Deuterium, is a stable isotope and thus produces no radioactivity. The only threat it could pose is flammability, because chemically, Deuterium reacts like hydrogen. But this property is not a big problem, since reasonable techniques for storing hydrogen have already been developed. Another option, as has already been mentioned, would be to store Deuterium as water and separate it from oxygen at the reaction cite1,2. This option would require very simple means of containment to eliminate the flammability risk. During the course of reaction, Deuterium would only be used in small amounts, in a vacuum chamber devoid of oxygen, which makes explosion impossible. However, because of the small atomic sizes of Deuterium and Tritium, some minimal leakage is inevitable. While in case of Deuterium it is not a problem, there is some concern for Tritium. Tritium is a radioactive isotope and may increase radioactivity background in the area of leakage. But, if proper storage techniques are used, Tritium would only be able to escape at unnoticeable rates, without increasing radioactivity background above the norm, imposing no threat to the power plant workers. Also, Tritium would only need to be stored in minimum amounts in order to get the reaction started again in case of operation interruption. Most of the Tritium is going to be produced during the reaction inside the reactor from lithium5. In an extreme case, even if all the contained Tritium is let out of its storage as a result of a failure, its concentration in the atmosphere should contribute less radiation than the permissible level by the time it spreads to the plant’s fence3. Moreover, Tritium has a very short half-life of only 12 years, as compared to the materials used in fission reactors, for which the half-life approaches thousands of years2. This makes fusion fuel much safer than that of fission or even fossil fuel power plants.
Operation
There are a number of conditions that need to be satisfied in order to facilitate a fusion reaction. The reactants’ nuclei need to have enough kinetic energy (or, roughly speaking, speed) to overcome the electrostatic force, which causes the positively charged nuclei to repel. The combining nuclei need to get in the vicinity of each other where the strong nuclear force will overcome the electromagnetic force. In macroscopic terms, this means that the gas of the reacting material has to be heated to a certain temperature before fusion can occur. That temperature is on the order of 100 million degrees Kelvin2,6. At such conditions, the helium isotopes’ atoms become completely ionized and the state of mater (like solid, liquid, or gas), composed of separate ionized nuclei and electrons, is called plasma. As the plasma is capable of conducting electricity, it can be heated by inducing electric current in it and then confined in a magnetic field. This is the principle of operation of a tokamak. On a large scale, plasma confinement and heat isolation (to keep the temperature high enough for a long time) are the issues with which scientists and engineers are currently struggling. The number of conditions that have to be precisely met for reaction to occur—such as plasma temperature and pressure, fuel supply, reactants and plasma purity—is in fact what makes fusion reactors much safer than any other source of power.
In case of a reactor failure during operation, there should be little threat to either the environment, or, in most cases, the power plant itself. An operating fusion reactor is much safer than a fission one, because a critical amount of fuel is not required. There is only a small amount of fuel present in the reaction zone at any time, which makes a meltdown impossible3. Even though the plasma in an operating reactor is heated to a tremendous temperature, it is not very dense, and can easily cool to harmless temperatures. So, if a magnetic confinement mechanism fails, the plasma will come in contact with the walls of the reactor and cool, after which, the reaction will stop. Even if plasma harms the reactor, damage should only be caused to the inner surface of the toroidal chamber. The plasma, in any case, should not be able to melt through the reactor walls. Some replacement of the inner parts might be necessary, but an explosion is practically impossible. Firstly, since the reaction is conducted in vacuum, there is nothing for hydrogen to react with chemically after the plasma has cooled. Secondly, even if in some extreme case leakage of air into the reaction chamber occurs, the hydrogen isotope concentration there will be too small for an explosion. Also, once the plasma is colder than the required temperature, the reaction simply ceases and, therefore, no runaway nuclear reaction is possible, as opposed to the case of a fission power plant failure.
If the fuel supply mechanism fails, the reaction simply comes to an end as well. There is no danger if the magnetic field continues to operate in the absence of fuel. Fuel shut down can also be used as means of accident prevention if a failure is suspected. The case of fuel leakage has already been discussed and does not present a great threat. If the gas constituting plasma leaks out, concentration of Tritium in it should be minimal and essentially safe. As explained by the Institute of Physics Report, “Fusion power stations would present no opportunity for terrorists to cause widespread harm (no greater than a typical fossil-fuelled station) owing to the intrinsic safety of the technology”2. Thus, the risk of a fusion power plant failure is less than that for the majority of current sources of energy (i.e., plants operating on coal, natural gas, nuclear fission, etc.).
Waste
Finally, the waste products of fusion reaction are either much safer than those of other kinds of power plants, or are absolutely harmless. The discussed reaction of Deuterium with Tritium, as can be seen in Fig. 2, produces the regular isotope of helium and a neutron. It is the same isotope of helium that is used to fill air balloons which is not radioactive and cannot activate the equipment. However, the neutrons, although not radioactive themselves, are capable of activating the reactor’s structure, especially the metallic parts, when they hit them at high speed. This effect can be mitigated by using less reactive materials (carbon fiber has been proposed), which will produce short half-life waste. In contrast, even regular materials activated by high energy neutrons have a half-life of only about 30 years or less which is much less than the half-life of nuclear waste produced by fission. So, no complex storage would be required. Eventually, activated parts of the reactor will have to be replaced. This procedure will not have to be conducted frequently, as the parts should hold for approximately 40 to 50 years (the lifetime of a regular power plant of any kind)1,3. By the time replacement parts are needed, waste in the old activated parts should already degrade, and they should be safe to dispose of or recycle as metal3. Hence, fusion technology has a direct advantage over other sources of energy.
Conclusion
In the current state of rapid use of natural resources and depletion of easily available ones, there is a high demand for alternative sources of energy. Importance of this is also increasing in the light of need for ecology protection. Fusion power would be the best answer to the problem, as the fuel it requires is virtually unlimited and the waste it produces will not impose a negative impact on the environment. There are multiple other advantages in developing and transitioning to fusion as a source of energy, among which are intrinsic safety of stations’ operation, relative ease of resources acquisition and waste handling, and minimal costs associated with operation. The research in the field is rapidly growing and commercial use of fusion energy is not too far off.