M Dwarf Planetary Systems: Are They Habitable?
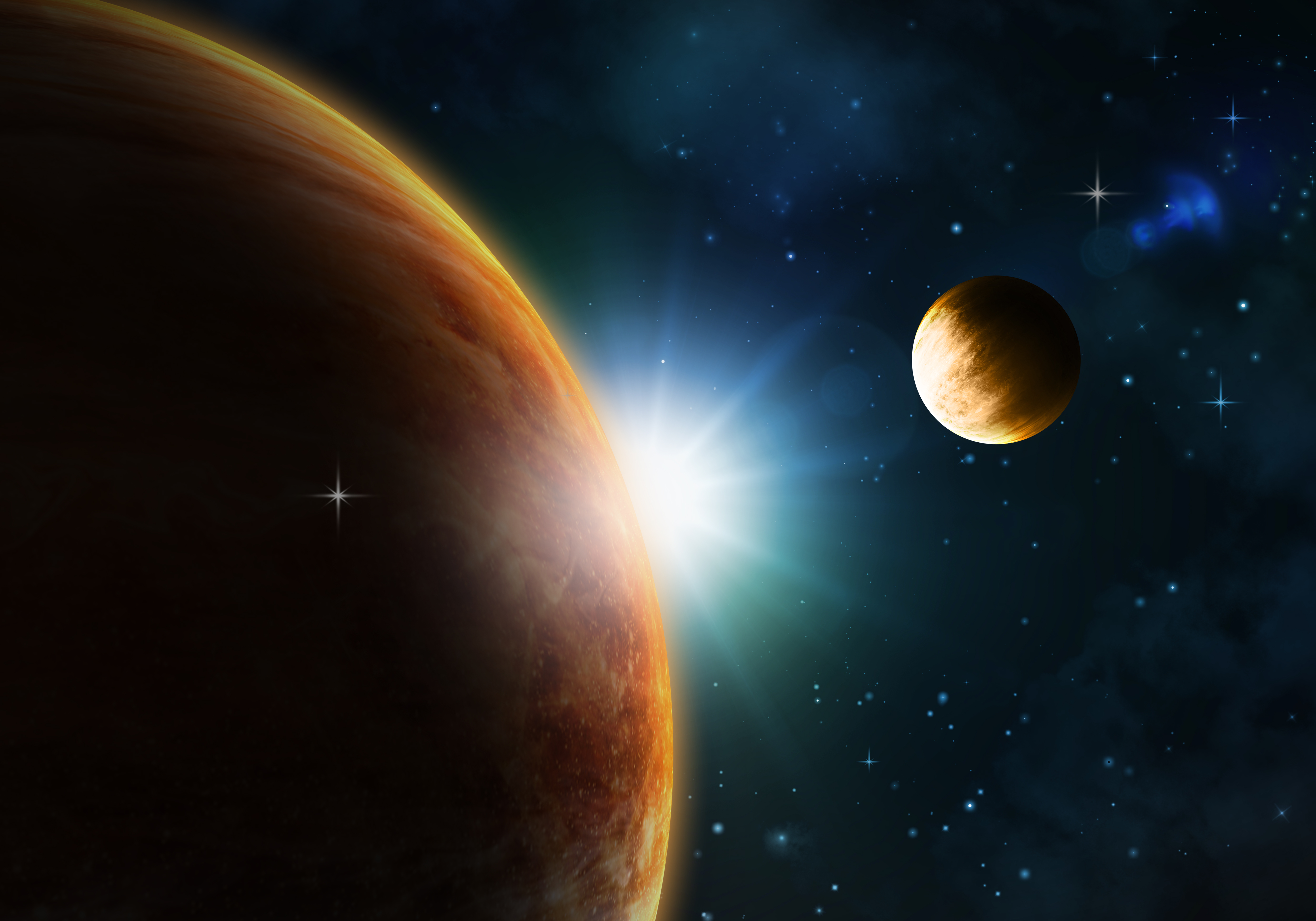
Alien life: Perhaps the greatest mystery of our time. Scientists have endeavored to find extraterrestrial life for decades, monitoring electromagnetic transmissions and searching for planets with the conditions necessary for harboring life. However, this search has been unsuccessful. Our galaxy is unfathomably vast, with billions of stars to investigate. Although this search may seem fruitless, surveys of the Milky Way have revealed a type of star that gives some astrobiologists hope, the M dwarf. As the most common classification of star in our galaxy (including our closest neighbor Proxima Centauri), M dwarfs are smaller and cooler than our Sun. Many of these stars are orbited by rocky planets, which have become prime candidates for the detection of extraterrestrial life.
However, these planets are subject to conditions much harsher than the conditions on Earth. Since M dwarfs are significantly smaller and cooler than the Sun, they irradiate light at longer wavelengths, peaking in the far-red (FR) range, shown in Figure 1. This could be hostile to life, specifically photosynthetic life, as it has been determined that the number of photons available for photosynthesis would be one order of magnitude lower on planets in M dwarf systems compared to the average terrestrial value. Furthermore, 40% of M dwarf systems host planets called super-Earths, with masses and planetary radii ranging from the size of Earth to the size of Neptune (Claudi et al. 2020). With greater mass comes greater gravitational acceleration, which may also prove detrimental to the development of life on these planets. Additionally, researchers have determined that the water content of these Earth-like planets orbiting M dwarf stars could be rare, creating desert planets unfit for a majority of life on Earth (Tian et al. 2015). Despite these potential hindrances, an analysis of the effects of irradiation, hypergravity, and intense aridity in M dwarf planetary systems on Earth-based life demonstrates that these distant planets may be able to harbor life after all.
To gauge the possibility of exotic photosynthesis occurring on planets orbiting M dwarf stars, researcher Riccardo Claudi of Italy’s National Institute for Astrophysics and his team created a study using different species of cyanobacteria (bacteria capable of photosynthesis). In particular, these cyanobacteria were chosen based on their unusual ability to use far-red light in photosynthesis. Chlorogloeopsis fritschii PCC 6912, Chroococcidiopsis thermalis PCC 7203, and Synechococcus PCC 7335 are capable of using far-red light in photosynthesis; Synechocystis sp. PCC served as a control organism, as it is not able to use far-red light in photosynthesis. The researchers then set out to simulate solar radiation, far-red radiation, and M dwarf-simulated radiation, using specific types of computer-controlled LEDs. This set-up acted as the illuminator, which, along with a spectrometer to measure the wavelengths of light being emitted, created a working stellar simulator. Underneath the stellar simulator was a steel incubator cell with atmospheric conditions comparable to Earth, where a Petri dish with the target organisms resided. Each of the cyanobacteria species tested were analyzed for a period of 240 hours, with photographic evidence available at the beginning of the experiment, 72 hours in, and 240 hours in, as seen in Figure 2 (Claudi et al. 2020, 12).
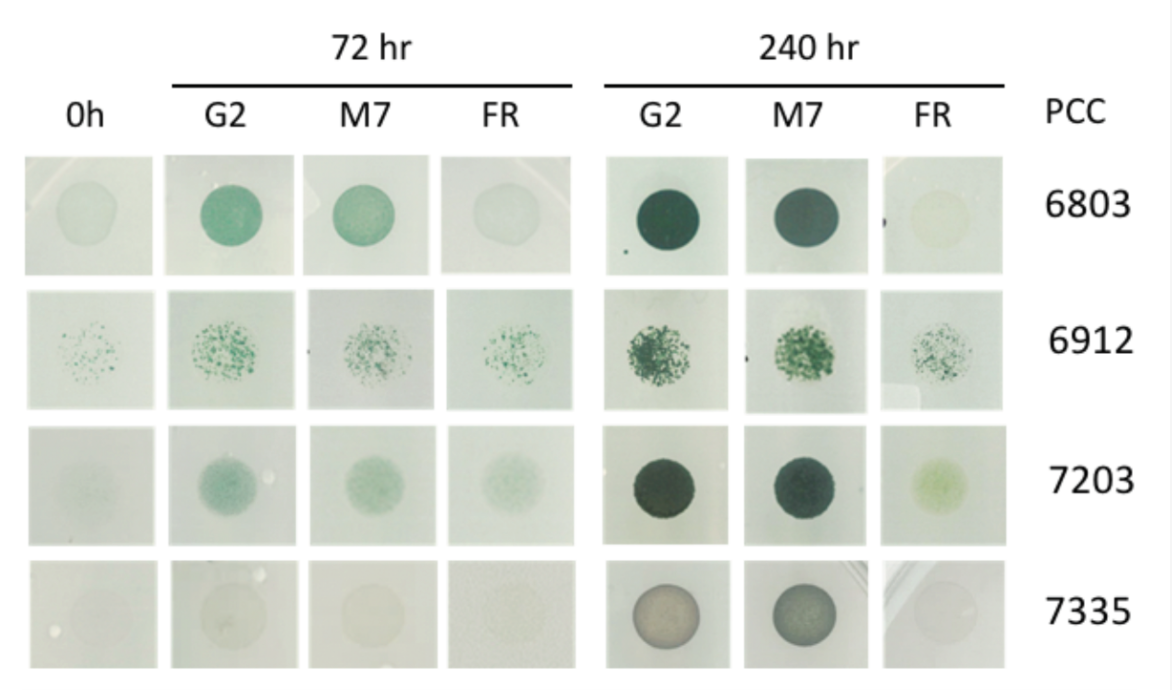
Figure 2: Claudi et al. 2020, Growth of Cyanobacteria Under Solar, Far-red, and M Dwarf Radiation
Note: G2 corresponds to the solar spectrum, M7 corresponds to the M dwarf spectrum, and FR corresponds to the far-red spectrum. The PCC (Pasteur Culture Collection of Cyanobacteria) number corresponds to the species of cyanobacteria, explained previously.
As Figure 2 shows, all species of cyanobacteria tested were able to grow under M dwarf-simulated irradiation, regardless of their ability to use far-red light in photosynthesis. This is because M dwarf spectra have both visible and FR components, despite peaking in the FR range. It is also evident that cyanobacteria species such as PCC 6803, which are unable to use FR light, are able to grow and photosynthesize by only using the visible part of the M dwarf spectrum. Additionally, the fact that these cyanobacteria can not only grow, but also photosynthesize in M dwarf-simulated radiation, greatly supports the hypothesized habitability of M dwarf planetary systems, as the presence of oxygen seems to be the most important feature to revealing life on other planets (Claudi et al. 2020). As such, all of the evidence from this study supports the habitability of M dwarf planetary systems, as all Earth-based life tested were able to survive the radiation conditions present in these systems.
Radiation is not the only hurdle to habitability in these systems; the intense gravity present on planets orbiting M dwarf starsis another essential factor. To examine the effects of hyper gravity on Earth-based life, researcher Shigeru Deguchi of the Japan Agency for Marine-Earth Science and Technology and his team used a variety of microorganisms, including the bacterial Paracoccus denitrificans, Escherichia coli, Shewanella amazonensis, Lactobacillus delbrueckii subsp. delbrueckii, and the eukaryotic Saccharomyces cerevisiae. Each of the organisms studied were placed in a tube containing a growth medium that was placed in a centrifuge, which spun at high speeds to create large accelerations. To measure the organisms’ growth over time, the centrifuge tubes were taken out and redispersed on a vortex mixer, with the number of live cells counted using the spread plate technique (Deguchi et al. 2011). The growth curves of each of these microbe species are illustrated in Figure 3.
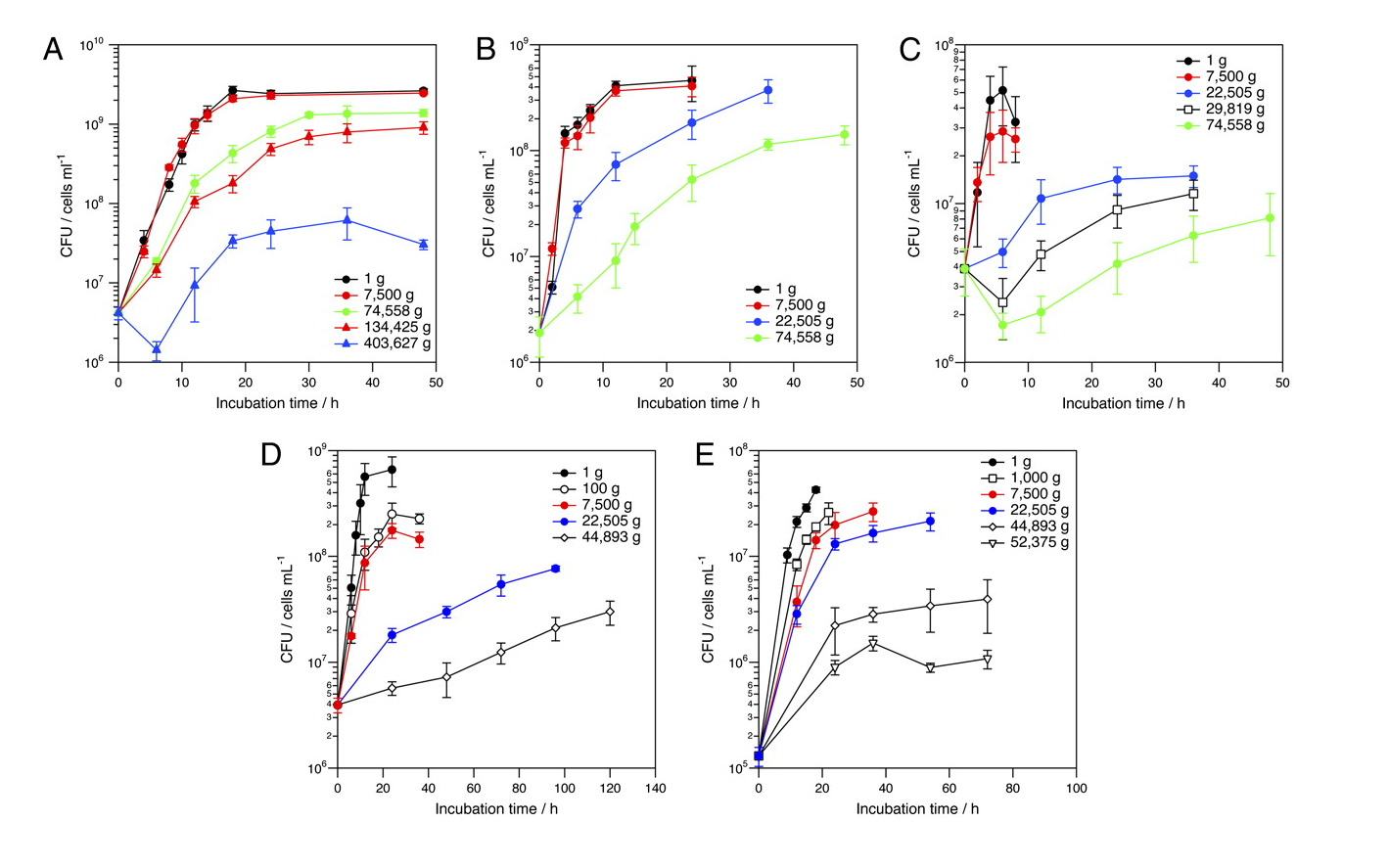
Figure 3: Deguchi et al. 2011, Growth of Various Microorganisms Under Hyperaccelerations
Note: A corresponds to P. denitrificans, B corresponds to E. coli, C corresponds to S. amazonensis, D corresponds to L. delbrueckii subsp. delbrueckii, and E corresponds to S. cerevisiae.
Figure 3 shows that all organisms tested were able to grow and reproduce under accelerations thousands of times greater than the gravitational acceleration on the surface of Earth. Due to the limitations of the experiment’s centrifuge, the researchers were not able to determine the upper limit of acceleration for some of the most tolerant organisms, including P. denitrificans, which was able to survive at an acceleration of 403,627 x g (Deguchi et al. 2011). Regarding the surface gravities of the aforementioned super-Earths, they are predicted to have surface accelerations significantly larger than Earth, but nowhere near the peak level that the microbes survived. For example, a planet orbiting an M dwarf star with a mass five times greater than that of the Earth is expected to have a surface acceleration of 25 m/s2, or about 2.6 x g (Claudi et al., 2020). With this in mind, the microbial results from this study negate hypergravity as an issue, as life overcomes yet another hurdle to habitability in M dwarf planetary systems.
The final effect that must be addressed when discussing the habitability of these planetary systems are drought conditions, which have been hypothesized to be present on these worlds due to their rare water content. To analyze this, lead researcher at the University of Göttingen Dominik Patzelt conducted a study to investigate the habitability of the driest non-polar desert on Earth, the Atacama Desert in Northern Chile. Some parts of this desert are so arid, no plant or animal life can survive. In this study, hyper-arid soil samples were collected from ten sites within the Atacama Desert, then suspended in either Z-8 media or sterile water. The soil suspensions were then transferred onto agar-solidified media, where the plates were checked regularly for bacterial growth (Patzelt et al. 2014). Figure 4 displays the results from these soil samples.
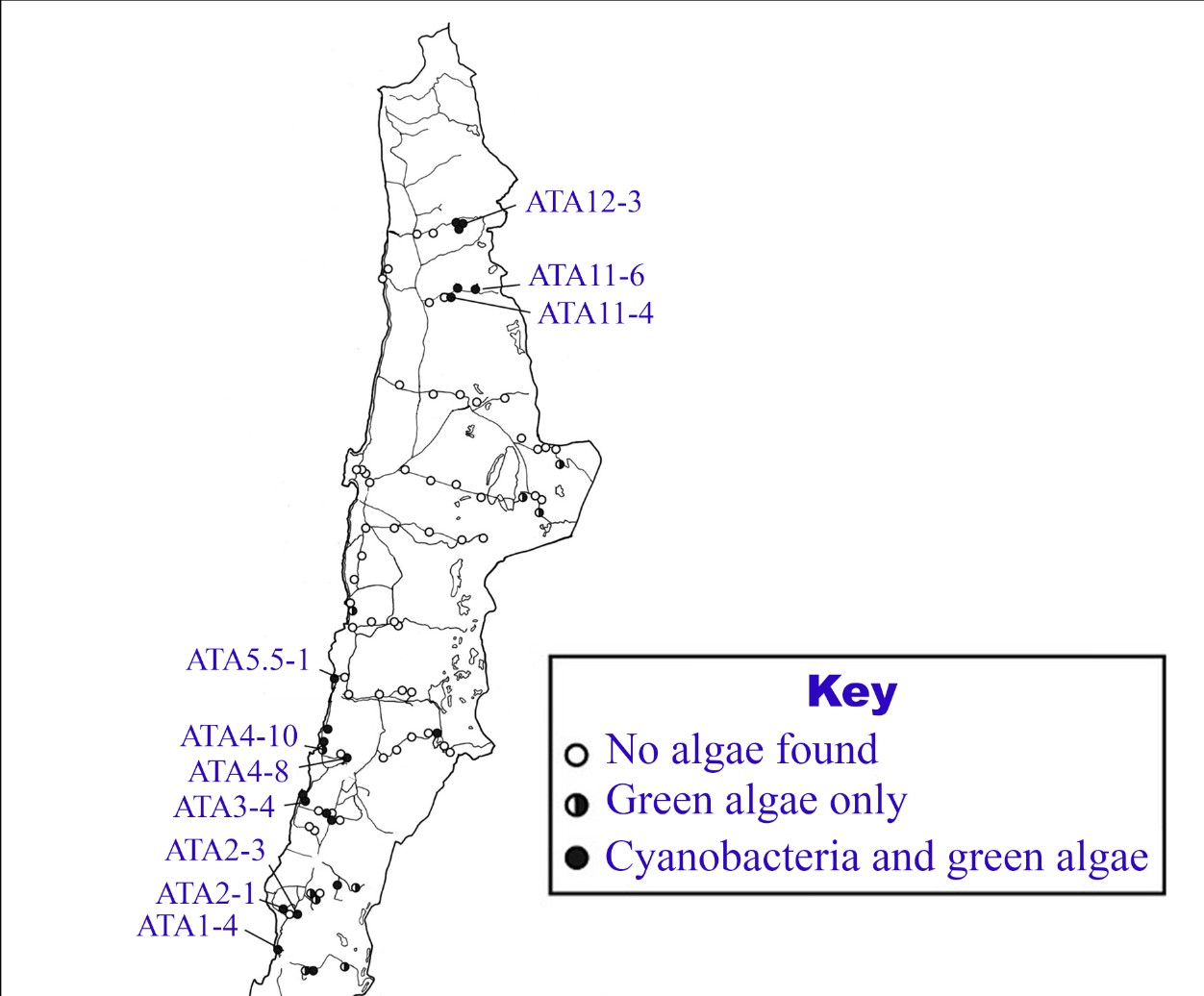
Figure 4: Patzelt et al. 2014, Map of Atacama Desert Including Sites Sampled
Note: Each of the codes (“ATA12-3”, “ATA11-6”, etc.) corresponds to a sample site. Only sites included in this study are labeled.
As Figure 4 shows, cyanobacteria were detected in all ten Atacama sites used in this study. Additionally, sites ATA4-8, ATA4-10, ATA 5.5-1, ATA11-4, and ATA12-3 had no vascular plants visible, suggesting the hyper-aridity of the soil, but were still able to support cyanobacterial life (Patzelt et al. 2014). These results strongly support the habitability of M dwarf planetary systems, as life has been shown to be able to survive drought in extremely arid soil ecosystems, conditions likely to appear on planets orbiting M dwarf stars.
The results of these three studies reveal crucial information regarding the habitability of these planetary systems. Showing that Earth-based life is able to survive M dwarf stars’ radiation, their constituent planets’ hypergravity, and the drought conditions these planets likely have strongly fortifies the argument for the habitability of these widespread star systems. However, this is not a completely comprehensive overview of the factors affecting M dwarf planetary systems. One such factor that future work must address is the effect of extreme ultraviolet (XUV) radiation on Earth-based life. M dwarfs are characterized by their high stellar activity, releasing large amounts of XUV radiation through stellar flares. Though this is hypothesized to rapidly change the radiation environment on the surface of these planets, some researchers postulate that the presence of a thick atmosphere or strong magnetic field would allow these planets to become habitable. Additionally, organisms on these planets could develop DNA repair mechanisms or UV-protecting pigments, further supporting the habitability of these planets (Claudi et al. 2020).
The encouraging results of these studies, combined with the sheer number M dwarf planets in our galaxy, establish the strong likelihood of the habitability of these planets. Though, this reality begs the question: If these planets are supposedly habitable, and are so common in our galaxy, why don’t scientists have conclusive evidence of extraterrestrial life? This is precisely the issue presented in the Fermi paradox, as all logic points to the existence of alien life, despite there being no evidence for it. The answer may lie in our methods of detecting life, which is primarily done by searching for radio waves coming from intelligent civilizations. Many scientists, including Carl Sagan, have postulated that our lack of radio wave detection is due to the high probability that advanced civilizations destroy themselves (Krauthammer 2011). Evidence for this probability is reflected in our own human race, as we live in an age of nuclear weapons and lethal biologic agents, which, if fallen into the wrong hands, could mean the end of intelligent life on Earth. It is precisely for this reason that we, as human beings, need to practice good politics and conduct state affairs appropriately: so that we may live long enough to detect the life we have ceaselessly aimed to encounter.
References
Claudi, R., Alei, E., Battistuzzi, M., Cocola, L., Erculiani, M. S., Pozzer, A. C., Salasnich, B., Simionato, D., Squicciarini, V., Poletto, L., & La Rocca, N. (2020, December 24). Super-earths, M dwarfs, and photosynthetic organisms: Habitability in the lab. Life, 11(1), 10. https://doi.org/10.3390/life11010010.
Deguchi, S., Shimoshige, H., Tsudome, M., Mukai, S., Corkery, R. W., Ito, S., & Horikoshi, K. (2011, April 25). Microbial growth at hyperaccelerations up to 403,627 × g. Proceedings of the National Academy of Sciences, 108(19), 7997-8002. https://doi.org/10.1073/pnas.1018027108.
Krauthammer, C. (2011, December 29). Are we alone in the universe? The Washington Post. https://www.washingtonpost.com/opinions/are-we-alone-in-the-universe/2011/12/29/gIQA2wSOPP_story.html.
Patzelt, D. J., Hodač, L., Friedl, T., Pietrasiak, N., & Johansen, J. R. (2014, May 7). Biodiversity of soil cyanobacteria in the hyper-arid Atacama Desert, Chile. Journal of Phycology, 50(4), 698–710. https://doi.org/10.1111/jpy.12196.
Tian, F., & Ida, S. (2015, February 16). Water contents of Earth-mass planets around M dwarfs. Nature Geoscience, 8(3), 177–180. https://doi.org/10.1038/ngeo2372.